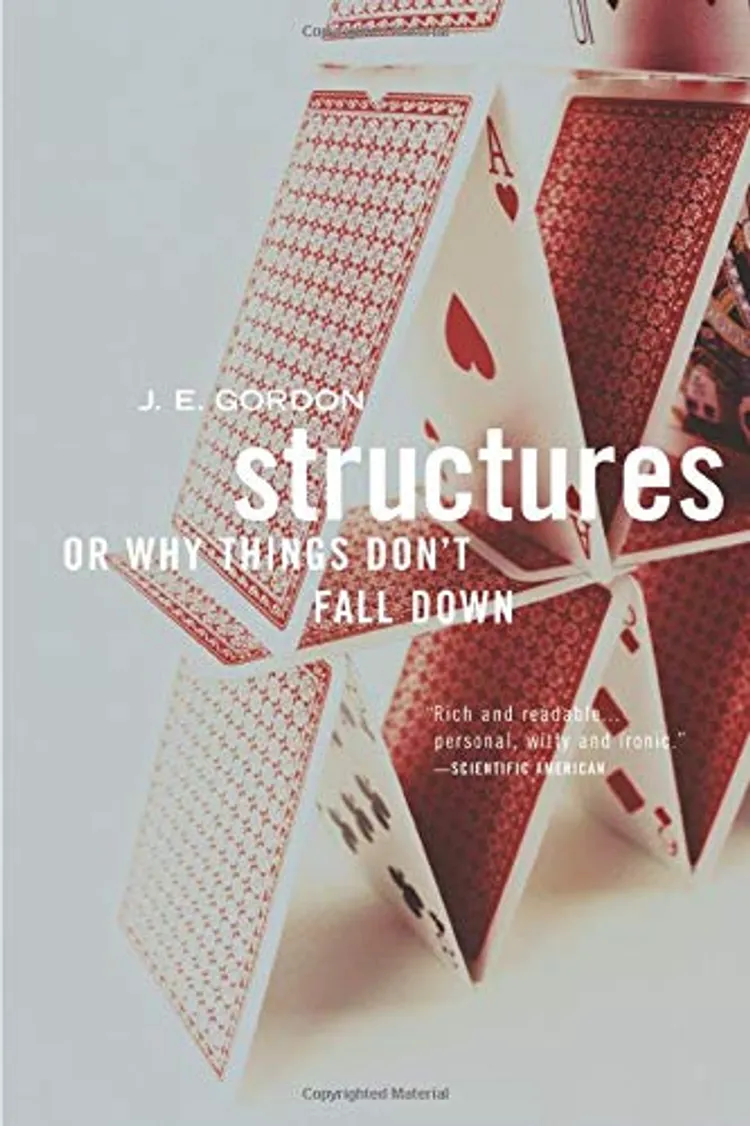
Structures
Or Why Things Don't Fall Down
by J. E. Gordon
🏆 Rated: 9/10
Summary
This book is an engaging exploration of structural engineering and the science behind why everday buildings, like bridges, roadways, or churches don't collapse in on themselves. As such it gives an interesting sneak peek into the world of civil engineering and covers the main concepts necessary to understand and answer the question of why things don't fall down.
The book delves into the fundamental ideas of engineering such as stress, strain, and different failure modes and properties of materials, revealing the intricate balance of forces that allows structures to exist without collapsing.
A structure, according to Gordon, is anything that can hold itself together against external forces, from bridges and buildings to bones and tree trunks. Structures as such are universal and the principles governing their organization are too. They are the same whether in human-made or natural systems and it is useful to know them if you ever want to build anything.
One of the main concepts in the book (and in structural engineering in general) is the relationship between stress (force per unit area) and strain (deformation caused by stress).
Stress:
Definition: Stress is the internal force per unit area that a material experiences when subjected to an external load. It is essentially a measure of how much force is being applied to a specific area of a material.
It is described by this equation:
Stress can come in different types:
- Tensile Stress: Pulls the material apart (like stretching a rubber band).
- Compressive Stress: Pushes the material together (like squashing a spring).
- Shear Stress: Forces that act parallel to the surface, causing deformation by sliding layers over each other (like scissors cutting paper).
Strain
Definition: Strain is the deformation or elongation that occurs in a material due to stress. It is a dimensionless quantity that describes the relative change in shape or size. It is essentially a measure of how much a material stretches, compresses, or deforms under stress.
Here and are the change in length and the initial length, respectively. In other words the more deformation the higher the strain.
The relationship between stress and strain is described by Hooke's law:
Here is Young's Modulus, a material specific property that we need to measure to find out. A Young's modulus determines how good or bad a material is at resisting stress.
Different materials can handle different kinds of stress differently well, which plays a huge role in which materials get used for which job. Often we mix different materials to get the best of both worlds, like concrete with iron bars embedded. Engineers calculate and manage the forces that act on a structure and then design the foundations etc. so that the structure can withstand the forces it will likely encounter with a healthy safety margin.
Gordon also explains different properties like elasticity, plasticity, and toughness and how they differ between materials like wood, steel, plastics or concrete. Microscopic structure informs most of these material properties. That is why people are working hard to discover novel materials for new purposes, which enables us to build cheaper, bigger, and better structures.
Structures also have all sorts of failure modes–the most common ones are fracture, buckling, creep, shear failure, corrosion, and fatigue.
- Fracture
- Definition: A material breaks into two or more pieces when the stress exceeds its strength. Different types include Brittle Fractures which occur with little or no plastic deformation (e.g., glass shattering). They are sudden and catastrophic and Ductile Fracture which involves significant plastic deformation before breaking (e.g., stretching of metal until it snaps).
- Example: A steel beam snapping under excessive tension.
- Buckling
- Definition: A structural member bends or collapses under compressive stress. Buckling is a geometric failure rather than material failure. Factors Influencing Buckling: Slenderness of the structure (long and thin elements are more prone), load direction and magnitude.
- Example: A thin column collapsing under a heavy load due to instability.
- Fatigue
- Definition: Repeated cyclic loading leads to the formation and growth of cracks, eventually causing failure.
- Example: Metal fatigue in airplane wings due to repeated stress from takeoff and landing.
- Creep
- Definition: Slow, permanent deformation of a material under constant stress over time, particularly at high temperatures.
- Example: Power plant turbines deforming under high heat and pressure.
- Shear Failure
- Definition: Layers of material slide past each other, leading to a loss of structural integrity.
- Example: Bolts shearing off under heavy loads.
- Corrosion and Environmental Effects
- Definition: Material degradation due to chemical reactions with the environment, such as rusting or erosion. Corrosion weakens the material, making it more susceptible to other failure modes like fracture or fatigue.
- Example: Steel bridges rusting and collapsing over time if not maintained.
The book also explains that often, modern engineering takes inspiration from naturally occuring structures, to save on material while creating stronger structures that can withhold the same or even bigger forces. A good example is the weblike structure of bones that gets replicated in some modern steel/concrete architectures.
Overall the book shows how engineers balance competing priorities, such as strength, weight, cost, and aesthetics, to create functional and durable structures.